News Story
Five Clark School Faculty Earn NSF CAREER Awards
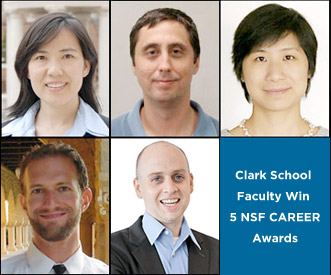
Top row: Dr. Baoxia Mi (left), Dr. Stanislav Stoliarov (center) and Dr. Dongxia Liu (right)
Bottom row: Dr. Michael Rotkowitz (left) and Dr. Christopher Jewell (center)
Five faculty members from the University of Maryland’s A. James Clark School of Engineering have been awarded National Science Foundation (NSF) Faculty Early Career Development (CAREER) Awards for their innovative research projects involving flame resistant material design and the conversion of methane into alternative fuels.
The NSF CAREER program supports the career development of outstanding junior faculty who most effectively integrate research and education within the goals and missions of their programs, departments, and schools.
Civil and Environmental Engineering Assistant Professor Baoxia Mi was selected for an NSF CAREER award for her proposal for "Graphene-enabled Synthesis and Surface Modification of Water Separation Membranes."
Mi, who also directs the University of Maryland's Membrane Innovation Lab, is researching the potentials of graphene oxide nanosheets for synthesizing a fundamentally new class of membranes and surface-modifying various existing membranes for high-performance water treatment.
According to Mi, membrane technology is generally considered one of the most effective strategies to tackle water scarcity worldwide; however, high energy requirements and long-term fouling issues have been major obstacles preventing widespread application of this technology. The graphene-based membrane technology that Mi proposed will hopefully be highly fouling-resistant, energy-efficient and capable of removing various contaminants from water.
Mi is optimistic that the graphene-based membrane technology will be very useful not only for drinking water purification and wastewater reuse, but also for renewable energy production, biomedical sensing and artificial organ development.
Assistant Professor of Fire Protection Engineering Stanislav I. Stoliarov was awarded a 5-year, $412,418 NSF CAREER Award for “Understanding Flammability of Charring Polymers.” His research project is focused on developing a quantitative understanding of burning for charring and intumescing polymeric systems. These systems represent one of the most promising and environmentally benign solutions to the hazards associated with polymer flammability. The mechanism of their flame resistance has not been well understood, hampering material development efforts.
This project will produce an in-depth understanding of char growth dynamics in a wide range of polymeric systems including a new generation of biodegradable materials. This understanding is expected to transform the field of flame resistant material design and enable qualitative improvements in public safety. The research results will be rapidly disseminated among scientists and practicing engineers and utilized to strengthen an array of partnerships with industry and professional organizations. This project will be used to foster students’ interest in science while promoting fire safety.
A proposal to use a new ceramic membrane to improve the conversion of methane into alternative fuels has also earned Assistant Professor of Chemical and Biomolecular Engineering Dongxia Liu a 5-year, $400,000 NSF CAREER award.
Methane, the main component of natural gas, can be converted into C2 (diatomic carbon)-based gases such as ethylene and ethane, which are used to produce commodity chemicals, polymers, and alternative fuels. It has the potential to play a significant role as a raw material for fuel and chemical production, reducing our need for petroleum.
As a resource, Liu says, methane is abundant and much cheaper than petroleum.
“The price of petroleum-like chemicals derived from methane is high because we do not have a cheap, efficient process for converting methane into these products,” she explains, “so we cannot use it directly as an energy source in the near future.”
As a result, she adds, a lot of methane goes to waste. “When we drill for oil, we also get methane, but most of the methane produced by oil rigs, especially in remote locations, is burned off instead of used. That pollutes our environment by releasing carbon dioxide into the atmosphere.”
Liu explains that the challenge to efficient methane conversion lies with the critical control of oxygen (O2) concentration and catalyst activity in the reaction chamber’s membrane, which stimulates the process.
The reactor’s membrane is responsible for both permeation, the process by which oxygen from the atmosphere is added to the reactor, and methane activation, the reaction that produces C2 gases. In an ideal situation, it would be capable of performing comparable levels of permeation and activation to ensure the speed and output required for industrial production of C2 products. Currently, no available membrane material can create the C2 gases as fast as it permeates O2. This leaves the catalyzed ethylene and ethane exposed to excess oxygen and vulnerable to the second reaction that converts them to CO and CO2.
Liu’s research group is developing a new type of catalytic membrane based on a biomineral called hydroxyapatite (HAP), a major component of teeth and bones. The new material is reactive-separative, meaning it is both a catalyst for the reactions and capable of permeating O2 in controlled concentrations into the reactor. Liu believes a perfected HAP-based membrane could perform each task at a comparable rate, wasting less methane, preventing the production of greenhouse gases, increasing product yield, and reducing the energy required to obtain the desired results. The technique used to create the membranes allows Liu’s team to control their structure at the nanoscale, so they can also be adjusted for different reaction conditions and products. The name of her NSF CAREER Award project is Surface Crystallization of Reactive Oxygen Permeable Hydroxyapatite-based Membranes for Direct Methane Oxidative Conversion.
Assistant Professor Michael Rotkowitz (Electrical and Computer Engineering/Institute for Systems Research) is the recipient of a 2014 NSF CAREER Award for "Decentralization and Parsimony for Implementable Control of Massively Interconnected Systems." The five-year award is worth $400,000.
The advent of complex interconnected systems has created a need to design and analyze controllers that can observe information from only a small portion of a network but may ultimately affect a large portion of the network. This includes smart building management, multi-vehicle systems and convoys, irrigation networks, large array telescopes, and the power distribution grid.
Developing these kinds of controllers is a key challenge in many cyber-physical systems problems. Conventional controls analysis assumes that one centralized decision-maker can access all available measurements, and determine the usage of all possible means of actuation. Most methods of design and analysis are extremely fragile to this assumption, and break down when such centralization is not possible or is not desired, leading to the field of decentralized control.
There is currently an enormous disconnect in decentralized control between celebrated theoretical advances and the concepts that are used for implementation, or even for computation. This is true of both recent advances and more classical results. Rotkowitz’s project pursues the key reasons for this disconnect, along with other impending barriers to the systematic implementation of decentralized control theory, particularly those which will become disabling when applied to massive systems. It undertakes theoretical investigations targeted to advance the field in a manner from which those barriers can be eliminated, along with much farther-reaching benefits, further coupled with computational and algorithmic investigations designed to parlay past and future advances into enabling technologies for sensitive applications including those listed above.
Rotkowitz’s research will produce a novel synthesis of the theory and methods of parsimonious recovery, which has undergone dramatic recent developments, with both the classical results and modern advances in decentralized control. It will further broaden the applicability of elegant and useful aspects of optimization theory to classes of problems that are paramount for the main scope of the project. The fundamental advances pursued in optimization and estimation have the potential to be of use much more broadly and to impact many other fields. This project further seeks to make broad impacts outside of its primary domain through collaborations with industry and with experimentalists, and through the creation of software tools for widespread use by non-experts.
Fischell Department of Bioengineering Assistant Professor Christopher Jewell was selected for a five-year, $438,000 NSF CAREER award for his vaccine design and immunotherapy research.
Jewell, who also serves as principal investigator for the Jewell Research Lab, is exploring how different biomaterials—such as those present in many experimental vaccines and immunotherapy treatments—interact with the immune system. Jewell’s team is using a new approach to study how different structures, concentrations, and combinations of biomaterials affect the organization and function of lymph nodes—the tissues that control immune response. All vaccines must reach lymph nodes to be effective, so the results of their research could lead to new biomaterials that serve not only as carriers, but also as materials that help tune immune response to fight particular diseases.
The Jewell lab team studies polymeric carriers that are important to the vaccine field, as well as new materials they are testing as improved vaccines. Through this research, the team hopes to uncover the reasons why some polymers work effectively as vaccine carriers, while others do not. Additionally, Jewell and his team study how the effectiveness of traditional vaccines—those that do not feature polymeric carriers—compares with that of vaccines that harness these new materials.
Along with his contributions to the biomaterials vaccine field, Jewell was also recognized for his role in helping to launch two new bioengineering programs at Wheaton High School in Silver Spring, Md.
The first program, established for the Biosciences Academy at Wheaton, featured a three-week module through which nearly 70 high school students worked in partnership with Fischell graduate students and postdoctoral researchers, who served as mentors, to create lessons about vaccines and conduct experiments in the Jewell lab.
The second program, established for the Biomedical Magnet Program at Wheaton, provided a select group of 20 students with a yearlong opportunity to learn about the research process by focusing on a bioengineering topic of their choosing. Selected topics include the use of stem cells to treat brain damage, therapies for multiple sclerosis, and biomechanics in sports medicine. Additionally, each program participant is partnered with a Fischell student or postdoctoral mentor, and tasked with interviewing experts, reading scientific literature and writing a review article. Each student will also develop a poster to be presented during a symposium in the Clark School’s Kim Engineering Building rotunda later this spring.
The goal of the program is to not only provide high school students with an opportunity to delve into bioengineering topics, but to also help students learn about science and engineering career opportunities, and to provide opportunities for research internships, Jewell said. Additionally, the program provides Fischell graduate students and postdoctoral researchers with an opportunity to gain invaluable scientific management and mentorship experience.
For more information about the NSF CAREER Awards program, visit: http://www.nsf.gov/funding/pgm_summ.jsp?pims_id=503214.
Also see: Two Clark School Faculty Members Receive Presidential Early Career Awards
Published December 16, 2013